Research on biomaterials is an interdisciplinary field that integrates subjects such as chemistry, biology, physics, and material engineering.
In our laboratory, we intend to expand the scope of research subjects in order to develop novel biomaterials, which can contribute to the biomedical field. Hereunder, I introduce some thesis topics undertaken in our laboratory.
USE OF POLYAMPHOLYTES AS A BIOMATERIAL

of the a-amino groups have been converted into carboxyl groups by SA addition.
Fig.1Carboxyl groups were introduced into e-Poly-L-lysine
Water constitutes 60% of the human body. Chemical reactions in living systems occur in aqueous solutions. Biopolymers such as proteins acquire unique and important characteristics in aqueous solutions.
Recent studies on polyampholytes, polymers with both positively and negatively charged groups, have focused on protein behavior, using polyampholytes as a model for proteins. In our laboratory, we are investigating the unique properties of polyampholytes and developing new biomaterials.
1. Cryoprotective properties of polyampholytes
Results of our research showed that several kinds of polyampholytes have a cryoprotective effect on cells in solution. This interesting phenomenon is characteristic of polymers with high electron charge, especially polyampholytes (Figures 1 and 2. Matsumura K. et al., Biomaterials 2009). Successful cryopreservation of many cell types, including human mesenchymal stem cells and induced pluripotent stem (iPS) cells, has been achieved (Matsumura K. et al., Cell Transplantation 2010; Matsumura K. et al., Cryobiology 2011).
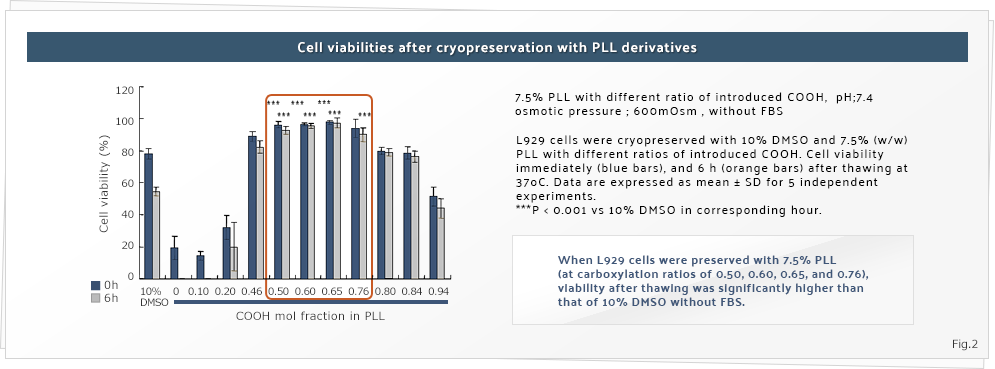

From the cryobiological viewpoint, cells are killed because of the damage caused by the intracellular crystallization of water during freezing. Therefore, a membrane-permeable chemical such as dimethyl sulfoxide is usually added in order to cryopreserve the cells. However, the cryoprotective effect of polymers such as polyampholytes that do not penetrate the membrane cannot be explained on the basis of the current knowledge in cryobiology. In order to understand the underlying mechanisms, we examine the interaction between the polymer and the polymer, the polymer and water, and the polymer and salts at low temperatures by using solid-state nuclear magnetic resonance (NMR). By using this method, we attempt to understand the mechanisms underlying the effects of polyampholytes on the extracellular environment during freezing.
The residual water content of several aqueous polymer solutions during freezing is shown in Figure 3. The polyampholyte (carboxylated poly-L-lysine) did not have the highest residual water content at low temperatures. This observation suggests that the residual water content during freezing might not explain its cryoprotective properties. Next, we used 23Na-NMR to examine the interaction between the polymer chains and sodium ions at low temperatures. The results showed that the sodium signal in the polyampholyte solution decreased at low temperatures; this observation suggests that the polyampholyte chains trap the sodium ions and restrain their movement at low temperatures. This phenomenon might decrease cell damage caused by an abrupt increase in osmotic pressure during freezing. In this manner, we are attempting to uncover the novel mechanisms underlying cryoprotective properties, from the viewpoint of materials science.
In our group, apart from COOH-PLL, we have also developed other cryoprotectants like dextran passed polyampholytes and synthetic polymers. We employ various monomeric systems and use RAFT polymerization to synthesize well-defined polymers with control over their molecular weights and structure. We are currently working towards develop newer synthetic polymer-based cryoprotectants with different architectures, by combining polymers with other macromolecules.
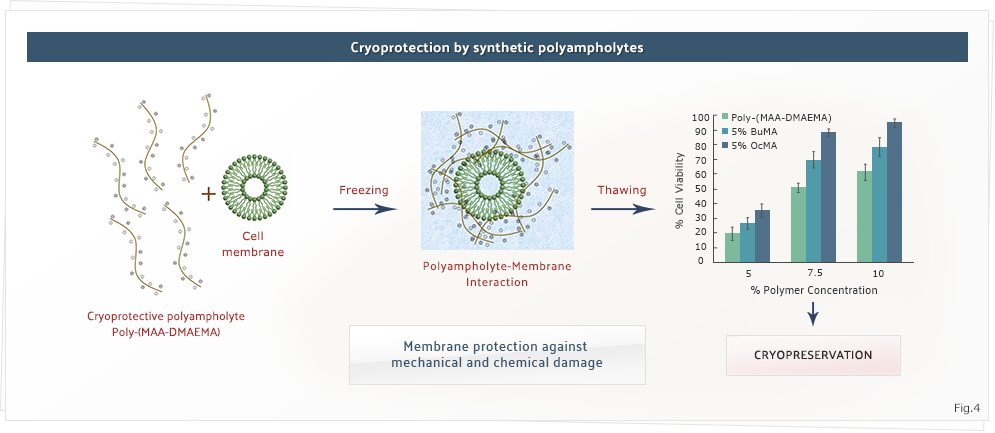
Recent Publications
- Robin Rajan, Minkle Jain and Kazuaki Matsumura Cryoprotective properties of completely synthetic polyampholytes via reversible addition-fragmentation chain transfer (RAFT) polymerization and the effects of hydrophobicity, Journal of Biomaterials Science, Polymer Edition, 2013, 24, 1767–1780.
- Minkle Jain, Robin Rajan, Suong-Hyu Hyon and Kazuaki Matsumura, Hydrogelation of dextran-based polyampholytes with cryoprotective properties via click chemistry, Biomaterials Science, 2014, 2, 308–317 (Selected as journal cover).
- Robin Rajan, Fumiaki Hayashi, Toshio Nagashima and Kazuaki Matsumura, Toward a Molecular Understanding of the Mechanism of Cryopreservation by Polyampholytes: Cell Membrane Interactions and Hydrophobicity, Biomacromolecules, 2016, 17, 1882–1893.
2. Application of polyampholytes as scaffold material
The application of biomaterials has extended from artificial organ development to regenerative medicine. Many studies are being performed on the control of proliferation and differentiation of stem cells when using various three-dimensional culture scaffolds by mimicking the biological environment. For example, we are attempting to develop a novel cell culture scaffold with cryoprotective properties by using polyampholyte hydrogel.
3. Elucidation of phase separation and its application
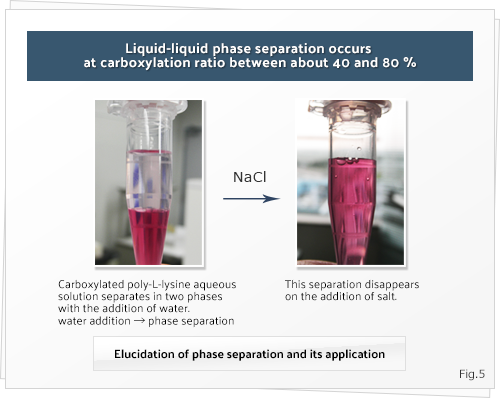
Carboxylated poly-L-lysine compounds (polyampholytes) exhibit lower critical solution temperature (LCST)-type phase separation to an extent that depends on their carboxylation ratios. Phase separation responds to changes in polymer and salt concentrations and exhibits temperature responsibility. A solution of this polyamplolyte exists in a single phase at high concentrations, but separates into 2 liquid-liquid phases on dilution with water. Because this separation disappears on the addition of salt, the phase separation could be ascribed to the polyion complex of the side chains of the polyampholyte (Figure 5). We are attempting to elucidate this phenomenon and apply it to novel biomaterials such as hydrogels and nano or micro particles.
4. Protein Aggregation Inhibition
Our group rationally designs zwitterionic polymers for the suppression/inhibition of protein aggregation. Most often, the polymers are synthesized by RAFT polymerization, ring opening polymerization or other synthetic routes involving organic synthesis. We have synthesized various polymeric systems including linear homopolymers, core-shell nanogels, hydrophobic polymers; and they displayed excellent propensity to protect proteins under extreme stress. We are currently working towards finding newer monomeric molecules and manipulating different polymeric architectures to achieve efficiency to match clinical needs.

Recent Publications
- Robin Rajan and Kazuaki Matsumura, A zwitterionic polymer as a novel inhibitor of protein aggregation, Journal of Materials Chemistry B, 2015,3, 5683-5689 (Selected as journal front cover and featured in Hot papers of 2015).
- Robin Rajan and Kazuaki Matsumura, Inhibition of protein aggregation by zwitterionic polymer-based core-shell nanogels, Scientific Reports, 2017, 7, 45777.
- Robin Rajan, Yu Suzuki, Kazuaki Matsumura, Zwitterionic Polymer Design that Inhibits Aggregation and Facilitates Insulin Refolding: Mechanistic Insights and Importance of Hydrophobicity, Macromolecular Bioscience, 2018, 18, 1800016.
- Neha Sharma, Robin Rajan, Sparsh Makhaik and Kazuaki Matsumura, A comparative study of protein aggregation arrest by zwitterionic polysulfobetaines: Using contrasting raft agents, ACS Omega (in press).
5. Stimuli-responsive polymers
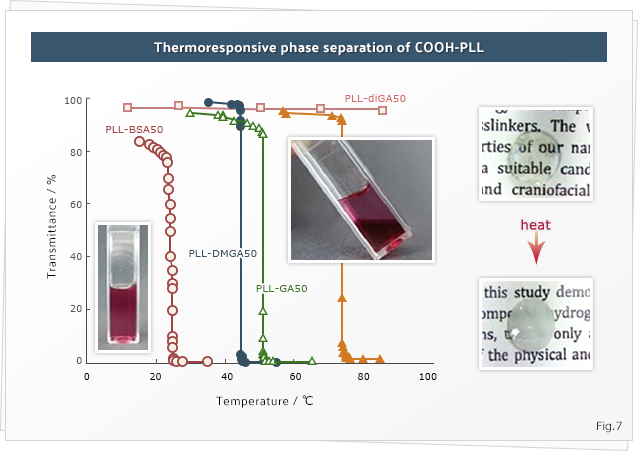
Stimuli‐responsive or smart polymers have attracted considerable interest in several fields, e.g., biomedicine, nanotechnology, and
numerous others, due to their versatility. Such systems exhibit an attractive feature, i.e., stimuli‐responsiveness, owing to their increased functionality which makes them suitable for applications in several fields. In our group, we are focusing on studying new thermoresponsive systems and are attempting to study the tuneability of such responses in order to employ them for various applications. We are also developing multi stimuli responsive systems to be used for biomedical applications.
Recent Publications
- Esha Das and Kazuaki Matsumura, Tunable phase-separation behavior of thermoresponsive polyampholytes through molecular design, Journal of Polymer Science Part A Polymer Chemistry, 2017, 55, 876-884.
- Robin Rajan and Kazuaki Matsumura, Tunable Dual-Thermoresponsive Core–Shell Nanogels Exhibiting UCST and LCST Behavior, Macromolecular Rapid Communications, 2017, 38, 1700478 (Selected as journal cover).
6. Development of freeze concentration as a next generation approach for delivery of nanomaterials
Intracellular delivery of macromolecules has recently attracted a great attention among researchers for application in biomedical and regenerative medicines. Previously, Electroporation, sonication approached were being used as a physical approach for delivery of therapeutics. However, parameters such as voltage shock and ultrasound irradiation could result into membrane defect or improper ionic balance which fails to perform cellular function and ultimately causes cell death. The safe and efficient delivery of biomolecules into cells is a major challenge in delivery system.
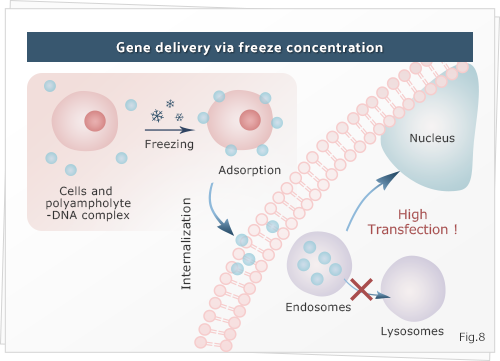
To find out alternative, our group has recently found the unique use of freeze concentration that facilitates the delivery of protein-nanocarrier complex. Freeze concentration is a physicochemical phenomenon wherein water molecules crystallizes to form ice which increasing the solute concentration in the remaining non-frozen system after phase separation during freezing. We found that on applying ultra-cold freezing -80 °C to the cells and proteins, the proteins are strongly adsorbed and internalized around the cell membrane. However, the use of a carrier or agents is needed to access their site of action in the cytoplasm or nucleus. Therefore, we also developed a new polyampholyte nano-carrier that possessed versatile properties such as surface charge, size and other physical properties for intracellular delivery by modifying ε-poly-L-lysine with an appropriate ratio of hydrophobic dodecylsuccinic anhydride and succinic anhydride. These polyampholytes were self-assembled by inter/intra-molecular electrostatic and hydrophobic interactions when the polyampholytes are dispersed into water.
In our study, the simple and effective combination of freeze concentration and polyampholyte nanocarrier was found for the use of in vitro immunotherapy and gene therapy applications. This simple, safe and cost effective technique was found to be a versatile method for delivery of model proteins and genes. We expect that freeze concentration approach can be applied to boost up antitumor immunity and could also show a great potential for efficient cell therapy or adoptive T-cell immunotherapy. We believe this technology may have broad application in nanomedicines.
Development of biomaterials compatible width living systems
We also perform basic and applied research on materials compatible with living systems; this research is aimed at restoring the function of living systems in tissue engineering. In particular, we are attempting to develop articular cartilage by using hydrogels with high mechanical properties (Figure 9). The research performed in our laboratory is aimed at combining chemistry and biology through the study of polymer chemistry and at developing materials that can control the function of living systems.

This laboratory was established in 2011.
We are looking forward to working with energetic and highly motivated participants.
Let's enjoy studying the interesting world of biomaterials !